Thursday, October 01, 2009
Tuesday, May 16, 2006
ideaccess

The idea is to use a (regular) 2-dim array of quantum dots (QD) to form nodal ponts in a conductive network. Each QD (node) is vertically connected to a bistable resonanant tunneling diode (formed in the "substrate"), and to a driving current injection line (bias line). Each node then forms a non-linear (bistable) element, and the network dynamics is governed by equations describing neural networks. The systems represents (in principle, at least) a direct implementation of nanoscale technology for computational purposes, without first building (nanoscale) transistors, logic gates, etc.
A Chip for a Neuron

The MIT Technology Review describes the research behind the first direct electrical interface between a semiconductor device and an individual mammalian nerve cell:
Context: The neurons of the mammal brain are hard to study, even when they're isolated in the lab. For more than a decade, scientists have analyzed the large neurons of leeches and snails by linking them directly to silicon chips that record their electrical activity. But mammalian neurons are smaller, and though they can be grown on silicon, the resulting signals are typically too weak to yield useful data. The electrical activity of mammalian brain cells can be read with electrodes, but that can be imprecise and requires careful preparation steps.Moritz Voelker and Peter Fromherz at the Max Planck Institute for Biochemistry have now designed the first computer chip that can record the firing of mammalian neurons, though so far only in a petri dish.
Methods and Results: As a neuron fires, the voltage across it changes, so a neuron on a chip affects how transistors underneath it conduct electricity. But in chips with conventional transistor designs, there's so much naturally occurring noise that it swamps neural signals. So Voelker and Fromherz changed the geometry of the transistors to suit the electrical properties of living neurons. They buried the conducting channels of their transistors a few nanometers deeper than usual, making the transistor more sensitive to the low voltages and firing speeds of neurons. The transistors could detect the signal of an ?individual rat neuron in a group, without the elaborate sample preparation that ?conventional electrodes require. What's more, the tran?sistors are significantly smaller than individual neurons and could in principle provide information on how subsections of a neuron behave.
Frequency dependent signal transfer in neuron transistors
Nerve cells are attached to open, metal-free gates of field-effect transistors submersed in electrolyte. The intracellular voltage is modulated by small ac signals from 0.1 Hz to 5000 Hz using a patch-clamp technique. The source-drain current is affected in amplitude and phase through a modulation of the extracellular voltage in the cleft between transistor and cell. The ac-signal transfer is evaluated on the basis of linear response theory. We use the model of a planar two-dimensional cable which consists of the core of an electrolyte sandwiched between the coats of a cell membrane and silicon dioxide of the transistor surface. Comparing experiment and model we obtain the resistances of core and coat, i.e., of the seal of cell and surface and of the attached membrane. The resistance of the membrane varies in different junctions. It may be lowered by two orders of magnitude as compared with the free membrane. This drop of the membrane resistance correlates with an enhancement of the seal resistance, i.e., with closer adhesion. The linear ac-transfer functions are used to compute the signal transfer of an action potential. The computed response is in good agreement with the observations of excited nerve cells on transistors.
©2006 wassem
N>T

The electrical coupling of randomly migrating neurons from rat explant brain-stem slice cultures to the gates of non-metallized field-effect transistors (FETs) has been investigated. The objective of our work is the precise interpretation of extracellular recorded signal shapes in comparison to the usual patch-clamp protocols to evaluate the possible use of the extracellular recording technique in electrophysiology. The neurons from our explant cultures exhibited strong voltage-gated potassium currents through the plasma membrane. With an improved noise level of the FET set-up, it was possible to record individual extracellular responses without any signal averaging. Cells were attached by patch-clamp pipettes in voltage-clamp mode and stimulated by voltage step pulses. The point contact model, which is the basic model used to describe electrical contact between cell and transistor, has been implemented in the electrical simulation program PSpice. Voltage and current recordings and compensation values from the patch-clamp measurement have been used as input data for the simulation circuit. Extracellular responses were identified as composed of capacitive current and active potassium current inputs into the adhesion region between the cell and transistor gate. We evaluated the extracellular signal shapes by comparing the capacitive and the slower potassium signal amplitudes. Differences in amplitudes were found, which were interpreted in previous work as enhanced conductance of the attached membrane compared to the average value of the cellular membrane. Our results suggest rather that additional effects like electrodiffusion, ion sensitivity of the sensors or more detailed electronic models for the small cleft between the cell and transistor should be included in the coupling model.
Tuesday, April 11, 2006
Axiology, Economy, Technology

Axiology, Economy, TechnologyWearable response to economic and axiological problems,
Many people would agree that the technological advances used to read the text on this screen and the way in which computers interact with one another are tools for achieving certain social ends; we sense that certain ends which are still distant, tacitly unspeakable, and for which a solution is still more or less ruled out (elimination of war, terrorism, illiteracy and endemic poverty...) will be sorted out thanks to these marvellous communication and data processing tools.
Nerve/Chip Hybrid

Nervy chip may open window into brain
Peter Weiss
In a feat that blurs the lines between science fiction and reality, researchers in Germany have combined living brain cells and semiconductor electronics in a single circuit.
Plastic posts on a microchip corral a snail neuron above a transistor.Fromherz
The new microdevice, which uses snail neurons because they are conveniently large, represents a step toward more-complex hybrid circuits, says Peter Fromherz, leader of the work. Such circuits would include up to hundreds of neurons and may enable neuroscientists to directly probe the physiological processes of memory and learning, he says. ..
Nervy chip may open window into brain
Peter Weiss
In a feat that blurs the lines between science fiction and reality, researchers in Germany have combined living brain cells and semiconductor electronics in a single circuit.
The new microdevice, which uses snail neurons because they are conveniently large, represents a step toward more-complex hybrid circuits, says Peter Fromherz, leader of the work. Such circuits would include up to hundreds of neurons and may enable neuroscientists to directly probe the physiological processes of memory and learning, he says.
"We want to make a biological neural network . . . and then supervise it, record [from] it, and look at what's going on," says Fromherz.
Finding ways of directly linking semiconductor components and cells also may further medical and technological applications, he notes. Teams in other labs are trying to meld brain tissue with microchips to make implants that can restore sight and computers that exploit nerve cells' information-processing abilities.
But those teams connect cells to circuits by impaling the cells with needle-like electrodes, Fromherz notes. That's how scientists recently commandeered a slice of a lamprey's brain to control a light-sensitive robot (SN: 11/11/00, p. 309).
Fromherz and Günther Zeck, both of the Max Planck Institute for Biochemistry in Munich, describe their snail-neuron-based circuit in the Aug. 28 Proceedings of the National Academy of Sciences.
To make their circuit, the duo patterned transistors onto a silicon wafer, placed snail neurons onto the surface, and then immersed the device in a cell-sustaining broth. The team also has begun experimenting with rat neurons. While those cells are smaller and harder to work with, they're more like human neurons than snail neurons are.
References:
Zeck, G., and P. Fromherz. 2001. Noninvasive neuroelectronic interfacing with synaptically connected snail neurons immobilized on a semiconductor chip. Proceedings of the National Academy of Sciences 98(Aug. 28):10457-10462. Abstract available at http://www.pnas.org/cgi/content/abstract/98/18/10457.
Further Readings:
Perkins, S. 2000. Lamprey cyborg sees the light and responds. Science News 158(Nov. 11):309. Available at http://www.sciencenews.org/20001111/fob4.asp.
Sources:
Peter FromherzDepartment of Membrane and NeurophysicsMax Planck Institute for BiochemistryD-82152 Martinsried, MunichGermany
Gunther ZeckDepartment of Membrane and NeurophysicsMax Planck Institute for BiochemistryD-82152 Martinsried, MunichGermany
Biomorphic Analog Pulse-Coupled Neural Circuits
These circuits are potentially useful for invariant pattern recognition.
NASA's Jet Propulsion Laboratory, Pasadena, California
Analog electronic circuits that operate with pulsed input and output signals are undergoing development. The pulsing behavior of these circuits is modeled after a similar behavior, called "spiking," that occurs in biological neural networks. In these circuits, the pulse times and/or the pulse-repetition rates can convey information. These circuits are intended especially for use in high-speed artificial neural networks, which, like the brains of animals that have vision, would process image data to effect invariant pattern recognition. (As used here, "invariant"signifies that the ability to recognize patterns would not be adversely affected by such effects as translation, rotation, distortion, changes in scale, or changes in brightness.)
Figure 1 depicts an example of input/output behavior according to one mathematical model of a biomorphic spiking neuron. Starting from the beginning of a pulse cycle, a membrane potential rises at rate that decays exponentially until the potential passes a time-varying threshold, at which point the neuron sends a spike along its axon. At the instant of the spike, the membrane potential returns to a resting level from which the cycle starts anew. If the threshold, the resting potential, or the rate of rise of the membrane potential is modulated, then the pulse-repetition rate (also called the "spiking frequency" or the "firing rate") of the neuron is changed.
By locally connecting neurons like this one into an array in which the axons of neighbors would transmit their spike trains via synapto-dendritic connections that would modulate the thresholds, one could construct a complex processing network. In a computational simulation, such a network has been shown to be capable of invariant mapping of binary patterns.
The invariance of the mapping is a result of encoding images in time rather than space. In particular, if the same image is fed as input to a different set of pixels but the same spatial relationships are maintained among parts of the image, the temporal representation of the image remains the same and the mapping is invariant to translation. Invariance with respect to brightness is achieved partly by recognizing that greater brightness is represented simply by a uniform increase in the average firing rates of all affected neurons.
The upper part of Figure 2 depicts a developmental spiking-neuron circuit. The clock voltage source (Vclk) pumps charge through a subthreshold biased transistor (M1) onto the gate capacitance of transistor M2, the gate potential of which represents the membrane potential. The current source constituted by the clock and M1 is intentionally made fairly poor (i.e., is made to have low resistance) in order to obtain a nonlinear buildup of membrane potential. When the membrane potential becomes high enough to pull M3 out of its linear current-vs.-voltage region, the voltage at the swing node rapidly decreases as M2 pulls the node toward ground. The low voltage on the swing node then triggers the inverter formed with M4 and M5 to go high, and the inverter potential is digitally buffered to the output terminal. The clocked switching transistor M7 latches the voltage output on the noncharging portion of the cycle of the current pump at M1. M8 and M9 are sized to constitute an inverter that triggers at a relatively high dc potential to insure an adequate spike amplitude before the discharge transistor M6 is activated. When M6 is switched on, all charge at the membrane is drained to ground (zero potential) or, alternatively, to a source of nonzero resting potential connected to the source terminal of M6. When the membrane potential falls, M2 shuts down and the swing node is pulled high again as M3 returns to its linear region. This change in the swing node returns the output to low, ending the spike and switching off the discharge transistor at M6.
The lower part of Figure 2 shows a synapto-dendritic input circuit connected to the swing node of a spiking neuron. In a locally connected network, there could be eight input circuits like this one for coupling the outputs from eight nearest-neighbor neurons as inputs to the affected neuron. Transistor Ms1 sets the gain of the coupling, while transistor Ms2 controls the timing. Essentially, the spike from a neighboring neuron injects charge onto the gate of Ms3 through Ms1. This charge then slowly leaks away via Ms2 to produce a decaying exponential current response through Ms3. This current modulates the threshold of the spiking neuron by pulling M3 closer to saturation, thereby enabling a decreased membrane potential to trigger a spike.
Figure 1. The Interval Between Spikes and the Buildup of Membrane Potential can be modulated by modulating the threshold potential.
Figure 2. These Analog Neural Circuits are designed to exhibit spiking behavior that approximates that of figure 1.
This work was done by Tyson Thomas of Caltech for NASA's Jet Propulsion Laboratory. For further information, access the Technical Support Package (TSP) free on-line at www.nasatech.com under the Electronic Components and Systems category.
NPO-20818
Download detailed Technical Support Package for this Brief
RAS: in-situ analysis in MOVPE
An analytical technique to be used in MOVPE must be applicable at pressures from 20 hPa up to atmospheric pressure. This rules out the standard methods of electron diffraction (LEED, RHEED) used in MBE, since they only function in ultrahigh vacuum (approx. 10-10hPa).
Instead, optical methods suggest themselves. The methods of reflectometry are particularly suited for the requirements of MOVPE since, on the one hand, information can be obtained from the bulk such as layer thickness and composition. On the other hand, minor modifications also make processes on the surface accessible whose knowledge is necessary for the fundamental understanding of the growth processes.
The reflectivity of a sample depends on its dielectric properties which, in turn, depend on the type of atoms and their arrangement with respect to each other. In this way, on the one hand, material-specific data can be obtained, such as the composition of a layer. On the other hand, the dielectricity is also temperature-dependent so that e.g. the exact growth temperature can be directly measured in situ. If heterostructures are deposited, the layer thickness and growth rate can be determined from the interface effects (Fabry-Perot oscillations).
Reflective anisotropy spectroscopy (RAS) provides information from the dependence of the reflected light on the polarization. To this end, particular crystal properties of the III-V semiconductors are utilized: The classical III-V semiconductors are known to crystallize in the zinc blende structure, a cubic structure. In these semiconductors, the reflectivity of the bulk is isotropic, i.e. the same everywhere in the material, and not dependent on polarization. This does not apply to the surface of the crystal because reconstructions are formed here as a result of symmetry breaking

Consequently, the surface reflects polarized light anisotropically. The spectra thus generated are also specific so that the surface reconstruction can be determined from them. This surface reconstruction or its modification during epitaxy permits conclusions to be drawn concerning the processes taking place during layer growth. This is important for the understanding of MOVPE and it also enables process control since it is thus possible to supervise the quality of the surface or dopings.
A RAS spectrum consists of a number of normalized intensity differences as a function of the wavelength and energy of the reflected light. The reflectivity of two crystal directions perpendicular to each other (on (100) surfaces, for example, [011] and [01-1]), is measured and the difference in intensities is divided by the intensity averaged over the two directions.
ISG1-IT

The section Ion Technology (ISG1-IT) is part of the Institute ISG1 "Semiconductor Thin Films and Devices". ISG1 is one of four institutes, which form the department ISG, working on the physics of thin films and interfaces. ISG1-IT is a Center of Competence for the application of ion beam techniques especially in the field of thin film modification and characterization. It is a founding member of the regional nanoelectronics collaborative research center "CNI - The Center for Nanoelectronic Systems in Information Technology". ISG1- IT is focussed on the topics:
Silicon based materials e.g. strained silicon, silicides
High-k dielectrics,
Ferroelectrics for memories and
Innovative Si based NanoMOSFETs, e.g. Schottky barrier MOSFETs.
ISG1-IT operates four different accelerators with very specific performance and possibilities. In addition, a number of thin film deposition setups, including MBE, CVD, PLD and sputter machines are part of our equipment, which is part of the extended and powerful installations of the entire department. This allows us to epitaxially grow or deposit a wide range of different thin films and to lithographically structure, modify and characterize planar structures or nanoelectronic devices.
Institute 1: Semiconductor Thin Films and Devices

Scientific Program
Ion Technology
Semiconductor Films and Nanostructures
The institute investigates fundamental problems in semiconductor physics and in semiconductor materials.
In the device development alternative concepts are explored and property limits are explored.
The epitaxy of classical III/V compounds and of GaN is a broad activity. Electronic and optical properties of the grown layers are measured.
With the grown semiconductor layer systems devices are developed to explore e. g. the maximum transistor frequency and the minimum transistor cross section.
Resonant tunnel transistors are investigated to study the quantum mechanical limit in the smallest electronic devices.
With the standard semiconductor silicon vertical MOSFETs of 30 nm gate length are developed following different concepts.
Hybrid devices combining superconductor and semiconductor device physics are studied to get to devices with unusual properties.
Monday, March 13, 2006
Transistor Probes Local Potassium Conductances in the Adhesion Region of Cultured Rat Hippocampal Neurons
Previous Article Next Article
The Journal of Neuroscience, August 15, 1999, 19(16):6767-6773
This Article
Abstract
Full Text (PDF)
Alert me when this article is cited
Alert me if a correction is posted
Citation Map
Services
Email this article to a friend
Similar articles in this journal
Similar articles in ISI Web of Science
Similar articles in PubMed
Alert me to new issues of the journal
Download to citation manager
Cited by other online articles
Search for citing articles in: ISI Web of Science (25)
Google Scholar
Articles by Vassanelli, S.
Articles by Fromherz, P.
Articles citing this Article
PubMed
PubMed Citation
Articles by Vassanelli, S.
Articles by Fromherz, P.
Transistor Probes Local Potassium Conductances in the Adhesion Region of Cultured Rat Hippocampal Neurons Stefano Vassanelli and Peter Fromherz
Department of Membrane and Neurophysics, Max-Planck-Institute for Biochemistry, D-82152 Martinsried/München, Germany
Sunday, March 05, 2006
مشروع انشاء خلاية تقدم وظائف الكترونية
مقدمة:
يهدف المشروع إلى إيجاد آلية تخاطب بين الخلية الحية (العصبية) والذاكرة
الفيزيائية الآلية.
النقطة الاولى:
1- دراسة الاشارات العصبية ومقارنتها بالبديل الكهربائي المنطقي (1-0) .
2- ايجاد آلية تخاطب وسيطة.
3- انشاء وحدات توصيل الفيزيائية.
النقطة الثانية:
انشاء ذاكرة حية تحوي بيانات رقمية.
الدعم والتمويل:
مضى على العمل بمشروع الخلية الالكترونية هذا أكثر عام بجهودنا وإمكانياتنا
الفردية، ودون أي دعم من جهات رسمية أو غير رسمية. ونتائج أبحاثنا ننشرها هنا
مجاناً مع السماح بالنسخ والإضافة شرط الإشارة إلى
الحقوق المحفوظة لنا.
وغني عن البيان أن توفر دعم لهذا المشروع سيمكننا من تطوير أبحاثنا بطريقة أكثر
فعالية.
هدف المشروع:
يهدف المشروع الى ايجاد بدائل حية عملية يمكن إدخالها في صناعة التكنولوجيا.
بحيث يمكننا الاستفادة من ميزات الخلية العصبية في الصناعة التكنولوجية. مثلاً:
- ايجاد موصلات بين العصب والآت. وهو ما يمكن أن يؤدي إلى إيجاد أعضاء اصطناعية
فعالة للإنسان المحتاج. وكذلك قد يمكن إيجاد ميزات إضافية.
- امكانية ربط الذاكرة الاصطناعية بحيث تتم معالجة المعلومات بواسطة الخلية
العصبية التي تتميز بـ:
1- توفير الطاقة، حيث لاتحتاج الخلية العصبية سوى 0,3 ميلي فولت.
2- سرعة المعالجة التي تتمتع بها الخلية العصبية.
3- إمكانية الخلية على تطوير ذاتها وإصلاح بعض أعطابها إذا وجدت في شروط صحيحة.
اين وصلنا:
على الرغم من ظروفنا التي أشرنا إليها أعلاه، خاصة لجهة غياب أي دعم، استطعنا
أن نصل حتى الآن إلى:
1- دراسة مفصلة عن الإشارة العصبية تبحث إيجاد حلول للتخاطب مع الإشارات
المنطقية النبضية (1-.).
2- إنشاء موصلات فيزيائية بين العصب والدارات الكهربائية.
3- إنشاء جهاز قياس يعمل بواسطة الكثافة النسبية لقياس الاستجابة الفعلية
للعصب.
4- بعض التجارب الحلية حول إرسال المعلومات للضفدع بعد تدمير المخ.
*- بعض الدراسات غير منشورة عل الانترنت بهدف الحفاظ على خصوصيتها خاصة أنه قيد
التطوير. ويمكن طلبها بالبريد الالكتروني على هذا العنوان:
جميع الحقوق محفوظة
وسيم الشيخ – سوريا :wassemshiek@hotmail.com – waseem shiek - syria
Wednesday, March 01, 2006
Cell-Transistor-Hybrid Systems
Cell-Transistor-Hybrid Systems
Cell-semiconductor hybrid systems have potential to become important for the discovery of new pharmaceuticals and for use as whole cell sensors in drug screening. In addition it is feasible that they will be the future building blocks of artificial neuronal networks and that they will play a key role in interfacing traumatized nerves. The interface between live cells and solid state physics is one of the key issues of this work and is an important topic of our research. In order to bio-functionalise a solid surface in a way that the cell can recognize it as an adequate partner we have developed a broad range of experimental techniques to both modify and analyse this interface. To avoid atrophy of the cells and to control the cell-substrate coupling the device surface can be modified by the deposition of ultrathin polymer films. Using this approach the exploration of the development and plasticity of electrical interactions between single cells is feasible by a convenient non-destructive method of maintaining long term electrical contact with cultured cells, at a large number of recording sites. This project will be focussing on various topics: for drug discovery using genetically modified cells as transducer elements for molecular signals, studying the spatio-temporal signal spreading in two-dimensional cell monolayers (cardiac myocytes), and building up two-dimensional neuronal networks.
Redefining Robots
Originally published in SMITHSONIAN (February 2000)
by Paul Trachtman
(Editor's note: An obscure philosopher once noted that "Science serves two purposes: to examine and explain the inexplicable; and to suggest the yet-to-be." At times these two directions converge, particularly when something is created that functions despite a lack of understanding how exactly it operates. Tunnel diodes are just one example.
In this excerpted story, an engineer at Los Alamos National Laboratories has created a new approach to robotics that has completely contravened the "accepted practice" in design. Despite naysayers, he continues building units that: a) are easily replicated and b) function. He is regularly castigated or, worse, ignored by academics in AI and robotics.
And yet these robots are functioning.... RM)
The sign taped to the door reads: "Please Don't Feed The Robots." Mark Tilden unlocks the door and leads me into his Los Alamos lab. He sets his laptop case on a table and walks straight to the barred windows where sunlight filters in on a large sandbox he calls his "Robot Jurassic Park." The white sand is full of tracks, and alive with several species of little solar-powered "bio-mechanical" insects. He watches as they sun themselves, wake up for short, random walks, and then doze off again to soak up more energy. A wire-legged bug, soldered together from tiny transistors, sensors and motors, with a solar cell stuck to its back, begins walking in one direction, then suddenly veers off in another, catching Tilden's eye. "When it makes a decision like that, what has just gone on inside its head?" he asks. "As the builder, I have to admit that even I don't know."
Saturday, February 25, 2006
Garnet Hertz - Experiments in Galvanism: Frog with Implanted Webserver
Overview
Experiments in Galvanism is
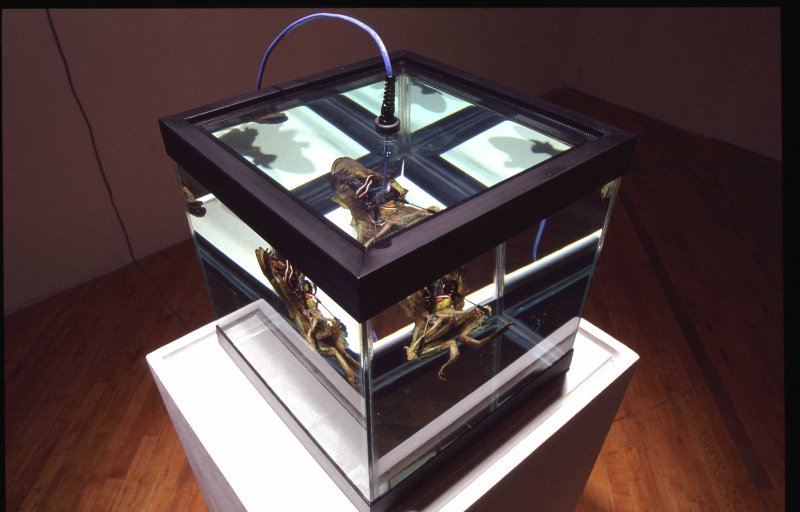
Garnet Hertz has implanted a miniature
webserver in the body of a frog specimen, which is suspended in a clear glass container of mineral oil, an inert liquid that does not conduct electricity. The frog is viewable on the Internet, and on the computer monitor across the room, through a webcam placed on the wall of the gallery. Through an Ethernet cable connected to the embedded webserver, remote viewers can trigger movement in either the right or left leg of the frog, thereby updating Luigi Galvani's original 1786 experiment causing the legs of a dead frog to twitch simply by touching muscles and nerves with metal.Experiments in Galvanism is both a reference to the origins of electricity, one of the earliest new media, and, through Galvani's discovery that bioelectric forces exist within living tissue, a nod to what many theorists and practitioners consider to be the new new media: bio(tech) art.- Sarah Cook and Steve Dietz (2005)
Neuron Transistor Photo (demo)
All this experiment for waseem shiek>>
NEURON TRANSISTORusing a leech ganglion, unites carbon with silicon. The nerve cell (green), about 80 microns wide, fires depending on the signals sent to the transistor. The fuzzy object piercing the nerve cell is a micromanipulator.



Neuron
Neuron
From Wikipedia, the free encyclopedia
Jump to: navigation, search
Drawing by Santiago Ramón y Cajal of cells in the pigeon cerebellum. (A) Denotes Purkinje cells, an example of a bipolar neuron. (B) Denotes granule cells which are multipolar.
Neurons (also spelled neurones or called nerve cells) are a major class of cells in the nervous system. In vertebrates, they are found in the brain, the spinal cord and in the nerves and ganglia of the peripheral nervous system, and their primary role is to process and transmit neural information. One important characteristic of neurons is that they have excitable membranes which allow them to generate and propagate electrical signals.
The concept of a neuron as the primary computational unit of the nervous system was devised by Spanish anatomist Santiago Ramón y Cajal. Cajal proposed that neurons were discrete cells which communicated with each other via specialized junctions. This became known as the Neuron Doctrine, one of the central tenets of modern neuroscience.
Contents[hide]
1 Anatomy and histology
2 Classes
3 Connectivity
4 Adaptations to carrying action potentials
5 Histology and internal structure
6 Challenges to the neuron doctrine
7 Neurons in the brain
8 See also
9 Sources
10 External links
The Biological Neuron

A neuron's dendritic tree is connected to a thousand neighbouring neurons. When one of those neurons fire, a positive or negative charge is received by one of the dendrites. The strengths of all the received charges are added together through the processes of spatial and temporal summation. Spatial summation occurs when several weak signals are converted into a single large one, while temporal summation converts a rapid series of weak pulses from one source into one large signal. The aggregate input is then passed to the soma (cell body). The soma and the enclosed nucleus don't play a significant role in the processing of incoming and outgoing data. Their primary function is to perform the continuous maintenance required to keep the neuron functional. The part of the soma that does concern itself with the signal is the axon hillock. If the aggregate input is greater than the axon hillock's threshold value, then the neuron fires, and an output signal is transmitted down the axon. The strength of the output is constant, regardless of whether the input was just above the threshold, or a hundred times as great. The output strength is unaffected by the many divisions in the axon; it reaches each terminal button with the same intensity it had at the axon hillock. This uniformity is critical in an analogue device such as a brain where small errors can snowball, and where error correction is more difficult than in a digital system.
Each terminal button is connected to other neurons across a small gap called a synapse [left]. The physical and neurochemical characteristics of each synapse determines the strength and polarity of the new input signal. This is where the brain is the most flexible, and the most vulnerable. Changing the constitution of various neuro- transmitter chemicals can increase or decrease the amount of stimulation that
